SAG mills are well established in the mining industry with several possible flowsheets featuring them. But these circuits consume significant amounts of energy and water. With alternative grinding options providing a means to decrease energy and water use, North American Mining asked a group of leading experts for their opinion of the future of these workhorses of the mineral processing world.
By Jonathan Rowland
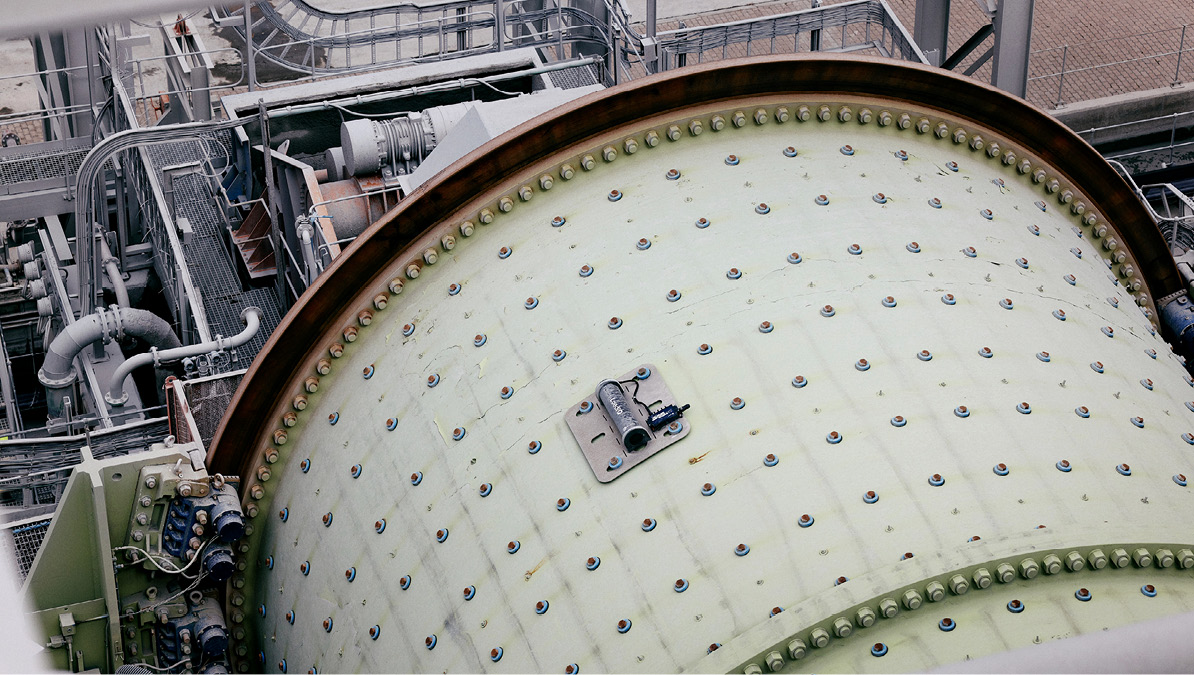
Grinding circuits lie at the heart of the minerals processing flowsheet. And at the heart of the grinding circuit is often the SAG mills. These mills have a long history in the mining industry and are thus well understood from both an operational, maintenance, and consumables (grinding media and mill liners) standpoint, with mines able to achieve high equipment availability and productivity.
“Since their early application in the 1960s, SAG mills have provided the modern mining industry with workhorses used for most high throughput grinding applications,” Bianca Foggiatto, director of Comminution and Processing at Ausenco, told North American Mining. “These mills can functionally replace most of the crushing equipment in a comminution circuit, resulting in a tight footprint and reducing common issues experienced in fine crushing plants.”
For all their benefits, however, these workhorses present challenges around energy consumption, water usage, and greenhouse gas emissions. As the mining increasingly looks to balance economic goals with lowering its environmental impact, it is worth asking: what is the future for SAG mills?
The energy challenge
It is widely recognized that “the grinding circuit requires the highest power demand within a minerals processing flowsheet,” said Garret Barthold, global product line manager for Horizontal Grinding Mills at FLSmidth. “SAG mills are particularly high consumers of energy and, as a result, there has been a push to improve their energy efficiency.” One example of this trend is the use of improved mill drive technologies, e.g., the use of gearless mill drives (for large SAG mills) or of large, geared drives with low synchronous motors and variable frequency drives.
“Changing the drive train technology on an existing mill may provide mechanical/electrical efficiency benefits,” explained the FLSmidth expert. “However, this type of modification is disruptive, costly, and often impractical in existing operations. Process optimization upgrades thus tend to be the most prominent avenue for sustainability improvements. These solutions include optimization of mill liner design and/or the implementation of sophisticated online mill load and charge impact monitoring packages, which help ensure the mill is utilizing the energy consumed in the most efficient way.”
“A subpar control system could lead to an up to 20% efficiency loss by failing to meet throughput or grind targets,” noted Rajiv Chandramohan, global technical director, Process Optimization and Debottlenecking, at Ausenco. “On the other hand, implementing effective process control solutions, such as advanced process control (APC) and model predictive control (MPC), enhances stability and efficiency in these circuits. Integrating advanced sensors for real-time ore characterization and wear rate monitoring can further boost circuit performance, as can the use of AI systems to dynamically adjust control parameters based on efficiency metrics like cost per ton and greenhouse gas emissions.”
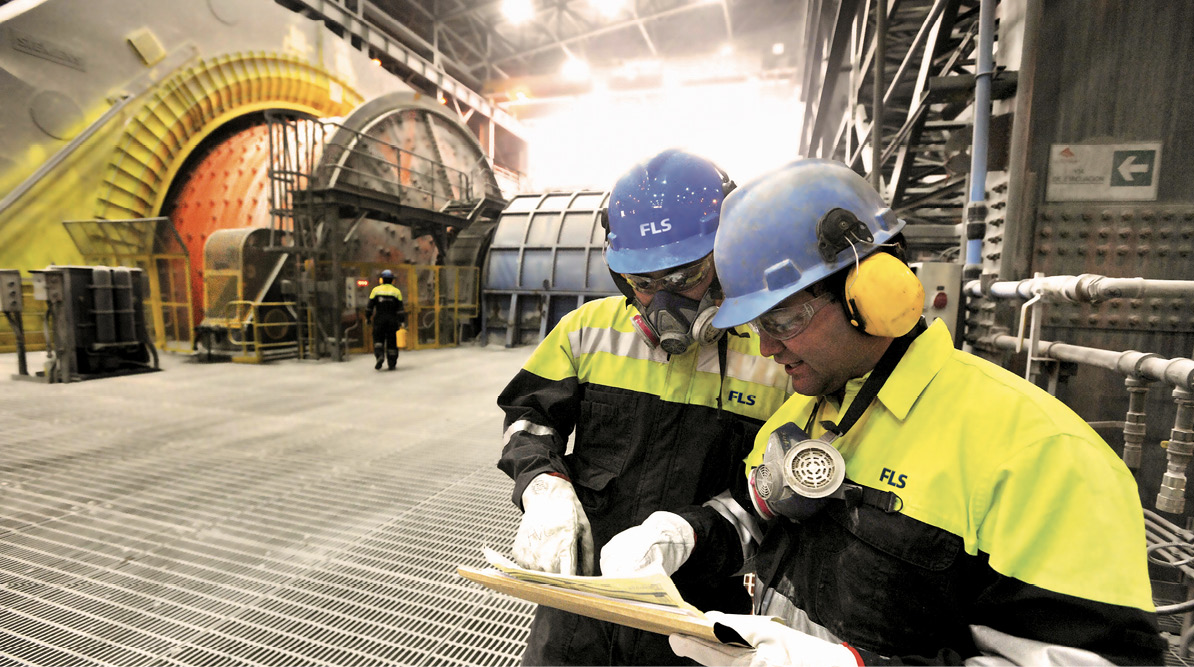
Indirect energy consumption: Grinding media and wear liners
Reducing indirect energy consumption (and costs) associated with grinding media and wear media consumption is an additional driver in comminution circuit flowsheet selection. As Ausenco’s Foggiatto explained, the production of media and wear liners requires mining, smelting, forging, and transport to site, with each stage contributing to GHG emissions. Indeed, in four to seven weeks of operation, a SAG-ball mill circuit can consume the same weight in steel used to construct the entire milling circuit structure.
Improving the wear life of mill liners is one way to tackle indirect energy consumption; it also improves mill productivity as downtime for liner replacement is reduced. Sam Hearn, global sales director at Multotec, explained how the company’s use of discrete element analysis (DEM) and finite element analysis (FEA) plays a key role when developing improved liner designs and formulations.
“DEM software enables us to simulate the interaction between the mill charge and the liners, and to evaluate the liner profile over the life of the liner,” Hearn said. “The analysis considers a range of variables, such as the ore’s bond work index, its specific gravity, the size of the grinding media, the mill speed, and the slurry density, allowing us to accurately model the performance and wear of our mill liners. This includes predicting the liners’ wear life to avoid unscheduled downtime and to extend the time between replacements.”
Emphasizing that no two mill liner applications are identical, this simulation “can guide very specific refinements in the liner design for each customer,” added the Multotec expert. “But a detailed understanding of the operating conditions is vital to ensure that the final solution delivers optimal results.”
Hearn concluded by saying that “the traditional use of steel liners in large SAG mills presents challenges. For instance, there may be bending stress inside the steel liner due to inexact fitting on the mill’s curved surface, and the higher rigidity of steel compared to rubber makes it less than optimal for absorbing the energy of material inside the mill. This is where composite liners come into their own, combining the impact resistance of Hardox 500 steel inserts or chrome-molly casting inserts with the absorption capacity of a specially formulated wear-resistant compound.”
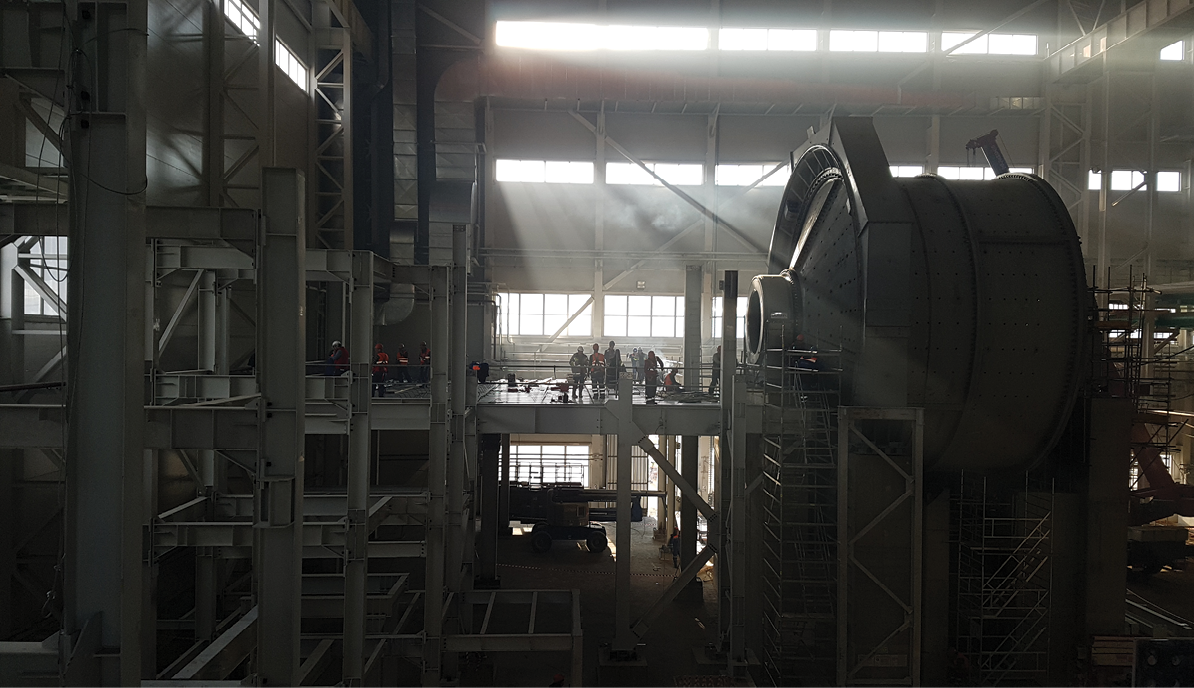
Alternatives to SAG mills: Going fully autogenous
SAG mill-based flowsheets typically have high greenhouse emissions (GHG) associated with two stages of grinding. This makes alternative flowsheets that include autogenous mills or single-stage grinding more attractive.
“Fully autogenous milling requires similar energy inputs as traditional SAG-based circuits, but it uses rocks as grinding media, reducing the indirect GHG emissions associated with grinding media usage,” explained Ausenco’s Foggiatto. “The selection of autogenous comminution technologies can thus improve project economics and, at the same time, reduce GHG emissions.”
“AG-pebble and AG-ball milling circuits are increasingly favored for reductions in media consumption, facilitating lower OPEX and reduced carbon intensity,” agreed Nick Green, vice president – Horizontal Mills at Metso. However, fully autogenous grinding is not appropriate for all ores. Ore testing, such as SMC and Allis-Chalmers media competency test “can provide some insight into whether an ore is amenable but pilot scale testing under the appropriate conditions is still the gold standard for designing AG milling circuits,” added Green’s colleague, Alan Boylston, director of Process Engineering at Metso.
Examples of AG-based circuits currently operating provided by Foggiatto include:
- Autogenous primary and secondary milling, e.g. Aitik copper-gold-silver mine in Sweden and Forrestania nickel mine in Australia.
- Single stage autogenous milling, e.g. Olympic Dam
polymetallic mine and Kambalda nickel mine, both in Australia. - Autogenous primary mills and secondary ball milling, e.g. Savage River iron ore in Australia and Ridgeway gold mine in Australia.
Alternatives to SAG mills: Dry comminution
When it comes to dry grinding flowsheets, all our experts pointed to the use of HPGRs, with Foggiatto describing them as “the most energy efficient circuit, although energy requirements for air classification can offset this benefit. Additionally, it has the potential to deliver ground products with narrower size distributions, which in turn can result in improved flotation performance and more effective tailings disposal with lower water consumption. It is not appropriate for all ores, but typically most favorable financially when ores are competent and power cost is high.”
HPGR circuits can “certainly operate with significantly lower energy than AG/SAG mill circuits,” agreed Green, although “HPGR and other dry comminution technologies are not universally applicable and often SAG mill operation is easier as it reduces material handling requirements.”
Table 1 shows a comparison of different grinding circuit flowsheets for a 4-million-tonnes-per-year copper concentrator designed to process moderate competence and hardness ores.
In addition to reduced energy consumption, dry grinding circuits can deliver a “a direct reduction in water utilization,” added Barthold of FLSmidth. “However, implementation of dry grinding is heavily influenced by downstream unit operations. For flowsheets where downstream separation and filtration are a wet process, conversion of the grinding circuit to a dry arrangement provides minimal benefit to overall plant water consumption. Conversely, dry grinding flowsheets provide significant water reduction benefits in applications with dry downstream minerals separation and refinement.”
“There is a perception that dry grinding reduces water consumption; however, where wet beneficiation is required for liberation, the net water addition is essentially the same, as water is just added later in the process,” affirmed Metso’s Boylston.
VRMs are another dry grinding technology. But whereas HGPR has already proven itself in mineral process applications, VRMs have yet to gain full acceptance. Yet the technology has a long and proven history in other applications, for example, the grinding of cement or granulated blast furnace slag. VRMs also produce a steeper particle size distribution, producing a feed well suited to flotation, while reducing waste in the form of ultra-fines and oversize and allowing for a good flotation size range.
Table 2 shows a comparison of the different approaches to design sustainable comminution circuits as compared to typical SAG-based flowsheets.
Innovative technologies: Improving SAG circuits
In addition to these alternative grinding technologies, there are “other new technologies currently being employed that improve energy efficiency and water consumption of processing plants,” continued Foggiatto. Importantly, these can be used in conjunction with existing SAG mills to improve energy efficiency.
“Technologies capable of ore/gangue separation at an earlier stage of the process unlock significant savings in energy and water consumption, as well as producing coarser tailings enabling safer disposal methods,” said Metso’s Boylston, picking up the point. “To examples here are bulk ore sorting and coarse particle flotation technologies.”
Bulk ore sorting (BOS): BOS is a low-capital and low-operating-cost preconcentration method that can effectively sort ore at high throughputs and reduce the amount of material that is ground to fine particles. The broad aim is to “take advantage of the natural spatial heterogeneity of an orebody to reject low grade material in smaller packet sizes and higher accuracy than current grade control strategies,” explained Ausenco Chief Technical Officer Matt Pyle. “The mineralogy of the deposit drives the sensor to be employed, which in turn determines the sorting technique. Depending on the BOS technology, the sensor is mounted on a conveyor or the mining fleet (such as a shovel.” Examples of BOS technology suppliers include Metso.
Coarse particle flotation (CPF): Conventional flotation “recovers a high proportion of the target mineral in a particle size range of 15 to 100 µm, but at finer (< 15 µm) and coarser (> 100 µm) particle sizes, recovery values are significantly lower,” said Pyle. “There are however technologies now coming to market for efficient coarse particle flotation. These provide the opportunity to coarsen the comminution circuit grind target or increase mill throughput, while producing coarse tailings for more straightforward dewatering and disposal.”
Examples of CPF technologies include Eriez’ HydroFloat, Jord International’s NovaCell, and the Reflux Flotation Cell and CoarseAIR, both from FLSmidth. Metso are also developing a novel flotation technology for CPF, which “can be applied to simple flowsheets, leading to further reduced water consumption and lower capital and operational costs,” added Boylston.
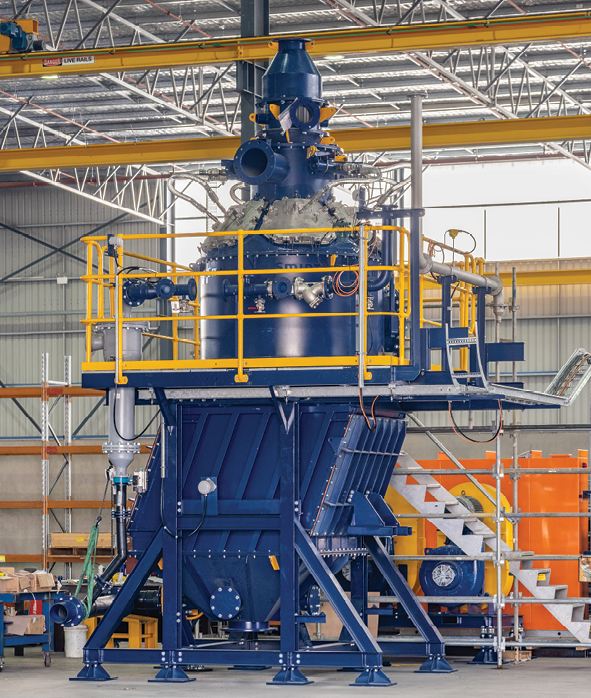
The future of SAG mills
In the immediate future, “SAG mills will certainly have their place in minerals processing flowsheets,” concluded FLSmidth’s Garret Barthold. “SAG mills are an established technology that allows for high capacity grinding through single machines. However, as we focus on grinding solutions that provide lower energy consumption and lower water utilization, dry processing solutions will gain more prevalence as mining companies implement and prove alternative flowsheets.”
“While alternative comminution flowsheets can deliver important reductions in energy, water consumption and GHG emissions, there are other novel approaches that deliver similar reductions by avoiding grinding of low-grade materials or targeting coarser grind sizes circuits,” added Ausenco’s Foggiatto. “It may therefore not be necessary to replace them to deliver a more sustainable project and SAG mill-based circuits may thus continue to be selected for new projects in conjunction with other innovative solutions, such as BOS and CPF.”