Evaluating An Embedded Monitoring Solution Based On The Industrial Internet Of Things (IIoT) And LoRaWAN Technologies To Keep Track Of Rollers’ Service Life.
By Ralf Luis de Moura, Alex Calegari Fracaroli, Danilo Bibancos, Eduardo Martinelli and Luiz Paulo Barreto
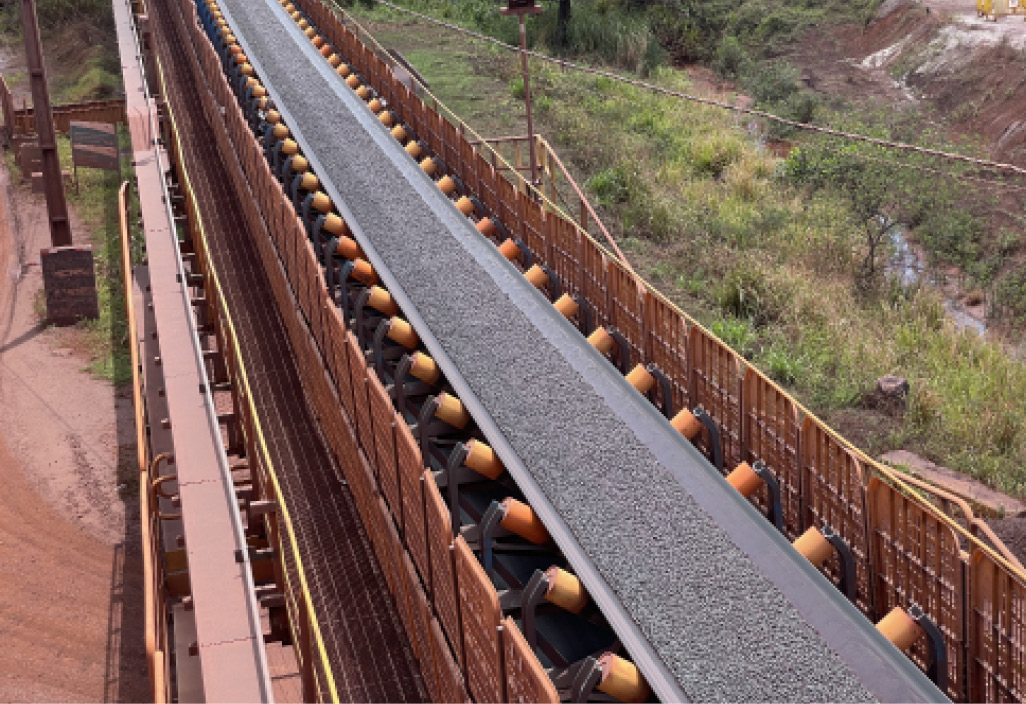
Abstract
Industry 4.0 is influencing the mining industry segment; from this influence, digital transformation projects arise by implementing new technologies to optimize production and maintenance processes. In the mining industry, the conveyor belt is one of the primary means of conveying large quantities of bulk material, capable of moving any solid volume over long distances at high production rates. Rollers are part of this process, and due to their massive use in conveyors, rollers’ failures may cause unexpected recurrent stops in operation and, consequently, impact negatively on operational effectiveness. Although several initiatives have been aimed at detecting, predicting, or preventing these failures, there are still no definitive solutions to prevent roller failures. This study has proposed and evaluated, in a case study, an embedded monitoring solution based on the Industrial Internet of Things (IIoT) and LoRaWAN technologies to keep track of the rollers’ service life. Findings show that embedded solutions may improve the fail detection capability and prevent damage, reducing unexpected plant shutdowns and production losses.
Introduction
Industry 4.0 and the Industrial Internet of Things (IIoT) influence several industry segments, including the mining sector. From this influence, digital transformation projects arise by implementing new technologies and differentiated processes to increase productivity, reduce waste, optimize production, and improve maintenance processes. However, none of this is achievable without solution sustainability.
The mining industry makes intensive use of conveyor belts as mechanisms for the continuous transport of materials in its production processes [1]. A conveyor belt is a traction component that transmits power and movement, transporting loads of different materials at different distances [2].
As with any industrial equipment, conveyor belts have failures [3], and these failures usually have adverse consequences for operations, especially in the mining industry. As they are the means of transporting materials, they can cause plant shutdowns when they fail, causing losses in production.
Several types of component failures can cause a belt to stop, including misalignment, wear, tears in the belts, and problems caused by the rollers that support the belts [2]. The mining industry relies primarily on periodic human inspection or online monitoring to detect component failures.
Several failures can cause a belt to stop [2]; over the years, several initiatives have been aimed at detecting, predicting, or preventing these failures. Although there are solutions for detecting tears, misalignment and wear, there is still no definitive solution to prevent roller failures.
Many components in a conveyor belt, particularly rollers, make it impossible to achieve the desired level of asset reliability-based only on human inspection. Over the years, several initiatives have been aimed at detecting, predicting, or preventing these component failures. Although there are solutions for detecting belt tears, misalignment and wear, there is still no definitive solution to detect and prevent roller failures.
When a roller reaches its life (prematurely or otherwise), it may damage the belt or even get on fire [3]. Its replacement is usually slow, and, in an emergency corrective situation, it can lead to several hours of production stoppage. Roller repair and replacement costs are a small portion of the overall operating expense (typically 10%-20% of the annualized component replacement costs of a conveyor) cost over a conveyor’s life, with belting being the highest cost. Therefore rollers, the smallest dollar items, put at risk the belt, the most important component on the asset.
The sheer quantity of rollers installed amplifies the problem. It is estimated that over 1,000 km of conveyor belts are upwards of 3 million rollers installed in Brazil. Assuming a replacement rate of 10% per year, mining operators have the impossible challenge of finding, every day, the roughly 1,000 rollers that will reach end-of-life amongst the universe of 3 million rollers installed. This problem is the equivalent of finding a needle in a haystack, and such a solution requires a system that can collect and manage enormous amounts of data.
Another problem is that rollers today are difficult to manage and control from the moment they arrive in the warehouse because they don’t have a single identification to identify and track. So, it’s not possible to identify batches coming from specific purchases to guarantee the expected SLA (Service Level Agreement), for instance, and, even though it’s possible to identify the batch and supplier, still it’s difficult to argue about capacity ranges due to the lack of any measurements from the core roller component (a roller may have been installed in a place where, for instance, a bigger impact capacity should be used, and that makes it wear out or burn much sooner than the expected life cycle).
Several attempts at automating roller inspection by substituting humans with robots, drones, or online monitoring. Such attempts are challenging due to the difficult terrain to be covered, often through tight spaces, and have low effectiveness as the frequency of inspection is still low. Technology is being developed to monitor rollers by placing external vibration and temperature sensors on their idler frames. Such sensors may still not bring the necessary precision to monitor and predict these elements’ useful life.
With the advent of the Industrial Internet of Things, miniaturization of electronic components, and the emergence of new communication protocols, it became possible to build an embedded solution capable of reading variables directly from the rollers and transmit that data over long distances with minimum energy consumption.
Typical Roller Failures
A conveyor system is a piece of mechanical handling equipment that moves materials from one location to another. Conveyors are especially useful in applications involving the transportation of bulk materials. Conveyor systems allow efficient transportation for a wide variety of materials, making them very popular in the mining industry [7].
Conveyor belts that suffer premature wear and need to be replaced frequently negatively impact productivity and increase the operating costs [6]. Rollers are critical components regarding the conveyor belt system [4], and the awareness of how rollers affect the conveyor and interact with other components is crucial. The idlers’ role is to provide load support to the conveyor belt with a low resistance to movement. Radial loading from belt and material is the principal requirement of most rollers and includes absorbing impact at the loading point and belt tension when located in curves [5].
The service life of rollers used to support belt conveyors depends on the appropriate sizing of their primary components (bearing, shaft, tube), the design quality of bearing seal kits, and the manufacturing processes utilized. Therefore, the analysis method to predict and extend the service life of rollers is also important to ensure the stable operation of the belt conveyor [2].
The leading causes of bearing failures are overload, bearing misalignment, incorrect assembly, improper storage, and seal system failure (contamination) [11]. If a problem arises in only one roller, there is a fire risk or that the sharp broken parts will cut the belt [13]. Over time, rollers deteriorate due to the wear process in which particles become trapped between the belt and roller to create abrasive wear under slight stress. Therefore, when a roller seizes as the result of a change in a rolling motion, the combined action of the belt and abrasive particles may transform the steel roller into a sharp instrument that can cut the belt [3].
The main factors that affect roller rotation include sealing and bearing lubrication problems, as the belt’s work environment is severe; in general, there is much dust on the site. The sealed form of bearing has a significant influence on the running flexibility for rollers. If not appropriately sealed, contaminant particles ingress into bearing results because of the roller rotation. Thereby, over time and with contamination, the bearing grease cannot play a proper lubricant role [2], making the roller unusable.
Defective rollers may provoke unexpected stoppages in production and affect the conveyor belt’s life and running resistance [2]. In the last instance, rollers may catch fire due to excessive heating during the operation.
Even though they are critical for the operation, rollers have evolved little in the past decades. The maintenance process still works reactively due to the difficulty of managing and controlling so many devices over such large geographical areas. Most mines do not have a single identification and tracking mechanism to address the problem. The tracking difficulty makes the tracking roller life cycle complicated, rendering the detection of replacement or maintenance needs impossible.
Enabling Technologies Within the Concept of IIoT
The advent of the Industrial Internet of Things (IIoT) opens opportunities to develop new forms of sensing and telemetry [14]. The miniaturization of electronic components and the emergence of LPWAN (long-power/long-range) communication protocols such as LoRaWAN made possible monitoring rollers in a low-cost and sustainable way.
Besides the traditionally cognitive inspections based on sound or vision [16]. There are available on the market various roller monitoring solutions, such as those described below:
- Sensors to collect temperature and/or acceleration at the conveyor belts support rollers’ anchor points, generating information in real-time for preventive maintenance analysis of the equipment. This solution uses BLE (Bluetooth Low Energy) to transmit the data to some gateway nearby (every 30 to 50 meters) that will resend it using WiFi, 3G/LTE, or LoRaWAN [15], [13].
- Solutions that use a typical fiber optic cable along the tracks with interrogator computing units at the end, and it is possible to obtain the roller’s temperature. A fiber optic solution detects changes from 1 (one degree), displaying alerts visually and audibly when identifying abnormal situations [12].
- A mobile robotic platform with manipulator arm and sensors, including a set of sensors including microphone, accelerometers, laser, and cameras, for the structural inspection of rollers [16].
- Thermal cameras embedded on drones or in a wire car may provide insights into abnormal roller temperatures in specific points of the conveyor belt segment.
All solutions presented, even cognitive inspections based on sound or vision, help detect and monitor rollers. Some solutions have a focus on disaster prevention or data collection for predictive actions. However, they all have one feature in common. They use indirect means to collect data or collect data from the support (idler frame) where the rollers are installed or parts close to the rollers. Although sufficient in some cases, the indirect collection does not show precisely the rollers’ internal problems that potentially cause failure.
Excess carry weight on the roller, for example, cannot be accurately identified by external sensors. Additionally, given the hostile environment, external sensors are prone to damage during routine operations in a mine, such as maintenance and/or cleaning procedures. Not mentioning the difficulties of providing energy for some of them.
Case Study
Iron ore is an abundant resource worldwide, with Brazil holding the fifth largest deposit, with 8.3% of its total reserves [8]. In the mining industry, much of the iron ore carried from the mines use conveyor belt systems. The use of this system usually reduces operating costs for the company. However, the maintenance costs are high due to the lack of knowledge of the rollers’ service life, making it impossible to optimize the planning of their replacement [9].
In order to increase operational reliability and reduce the need for corrective and preventive maintenance, and efficient system process monitoring is necessary, which should allow the detection of incipient failures [10].
A standard rubber impact roller was retrofitted with built-in sensors capable of detecting, in real-time, the smallest fluctuations of internal roller process parameters and transmitting them, via LoRaWAN, to the Plant Information Management System (PIMS) and a system in the cloud. Monitored parameters were: (i) left bearing temperature, (ii) right bearing temperature, (iii) rubber lagging/tube temperature, and (iv) load on the roller. All parameters were read and transmitted every 10 minutes.
Bearing Temperature
Bearing temperature is the most common form of roller inspection today and is indeed a critical determinant of roller life. Today, most mining rollers utilize ball bearings with permanent lubrication, making the health of the original bearing grease the most common limiting factor to bearing (and roller) life.
The life of bearing lubricants is highly susceptible to temperature. For every 15 °C (27 °F) above 70 °C (160 °F) up to the high-temperature limit (HTL), the relubrication period (or life of permanent grease) must be halved [17].
Because of bearing lubricant life, a roller with mechanical structure sized and bearing selected for 30,000 hours can have its expected life reduced to 15,000 hours operating at 85°C (185°F) or even to 7,500 hours if operating at 100°C (212°F).
The mining industry vastly recognizes this effect. Today, the most advanced maintenance inspection programs use thermographic cameras carried by inspectors to determine if a roller is operating above normal temperature conditions. Roller temperature inspection is also a popular method because it can foretell catastrophic roller failure through fire.
However, human or robotic inspection frequency is well below acceptable levels for reliable detection of critical conditions. Moreover, sensors that measure bearing temperature by indirect means, such as sensors placed on idler frames, present a low degree of accuracy. The separation between the sensor and the bearing allows too much heat dissipation, particularly in colder climates.
Therefore, the bearing temperature sensors were placed directly in contact with each bearing, lodged between bearing and shaft, providing the most reliable and accurate method for measuring bearing temperature.
Tube Temperature
The mining industry utilizes rubber lagging primarily on impact and returns rollers. The former reduces damage caused by material hitting the conveyor belt on transfer points, while the latter reduces the wear caused by material stuck to the belt grinding against rollers on the return side.
While fire-resistant rubber compounds have been developed for flammable applications (e.g., coal mining), such compounds’ wear life is significantly reduced compared to standard rubber. As such, rubber rollers are a common source of fire incidents in the mining industry.
Two primary sources of energy can create enough heat to initiate a fire on a rubber roller: (i) bearing temperature, which – whatever the cause – can propagate to the rubber, and (ii) rubber friction/compression force. While bearing temperature was already extensively monitored with independent sensors, rubber friction/compression needed to be addressed.
As such, an infrared temperature sensor was placed inside the roller, measuring the roller tube’s internal temperature, capturing the temperature of the rubber compound at its most critical point, the interface between rubber and steel.
Load
Conveyor belts are long-term assets, expected to last for decades in operation. Over time conveyor structures tend to deform. That effect is particularly pronounced on idler frames, which bend under load over time, causing misalignment and poor leveling between rollers.
When a particular set of idler frames is not leveled, the belt’s load is distributed unevenly across rollers, inevitably causing an overload on some. Such overload conditions can often cause excessive bearing misalignment. Under too heavy axial loads or misalignment, the bearing’s loading conditions can be such that the raceway no longer supports part of the contact ellipse. This phenomenon is called ellipse truncation, which leads to stress concentrations in the contact area. It may be detrimental for both bearing fatigue life and noise levels [18].
Therefore, a set of strain gauges was installed on the roller shaft to capture the actual load being forced onto each roller. Measurement of roller load was useful to determine conveyor structural alignment and a predictive tool to measure bearing health.
Built-In Electronics
Rollers operate in very harsh environments, filled with dust and ore slurry. Maintenance procedures call for periodic high-pressure washing of components multiple times per year, adding another layer of aggression to electronic components. Additionally, roll installation practices sometimes require operators to hit rollers with hammers and mallets to ensure proper fitting onto existing (sometimes old) idler frames. Such practices put at risk any external appendices to the roller structure, which can be damaged during installation.
As such, all electronics, in this case, were designed to be self-contained inside the roller, a completely sealed environment, protected from unintended user damage. It can’t contain external antennas or connection wires, for instance.
Another important fact is regarding energy needs for the circuit. As LoRa radio requires very little energy to send data (about 39 mA to send data and 11 mA to receive), [19] and can “sleep” when not in use, power needs are minimal. In fact, a roller’s spinning movement can easily be used to harvest the required power for the circuit, eliminating the need for batteries without any impact on the roll drag force.
Pricing Constraints
Rollers are a small portion of a belt conveyor’s overall operating expense, and their unit cost can be quite low.
As such, the embedded technology on each unit must be inexpensive, a fraction of the roll pricing levels. Current IIoT-focused electronic pricing already allows for the utilization of the technology on critical applications (i.e., impact beds, shiploads, stack-reclaimers, etc.) to justify a roll purchase. Additionally, the continued scaling of IIoT applications is rapidly reducing the cost of these components, such that the use of smart rollers tends to become ubiquitous shortly.
Unique Identification and Tracking
It’s important to have unique identifiers for each roller. This allows for tracking the rollers in the exact place they are and confirming the idler’s correct roller. With a unique identification, it’s possible to track the vendor and invoice from the batch purchase, and create automatic maintenance order against the exact roller, if something goes wrong, not mentioning being able to require free replacement costs if SLA covers them.
In the future, a small identification tag (QR code or Bar code) on the external side of the shaft or in the lateral part of the roller may be the only visible difference from regular rollers (even though this item is something possible and desired to be implemented in regular rollers as well).
Results
A set of 28 impact rollers, fitted with the described built-in electronics, were installed in four waves of tests and improvements over 16 months in a large iron ore mine in Brazil. Measurements were taken every 10 minutes and were transmitted to the Plant Information Management Systems and a cloud-based repository for processing and user consumption via the mobile app. Multiple improvements were made throughout the tests. All parameters measured (temperatures and loads) were accurate from the start, but multiple improvements had to be made on hardware reliability and antenna design.
Ultimately, an accurate and reliable solution was reached that showed the potential to:
- Aid on maintenance planning and reduce operational costs,
- Increase reliability and availability of conveyor belts,
- Avoid operational outages to change defective roller, Increase personnel safety and asset reliability, avoiding fire on rollers and conveyor belts,
- Reduce employee exposure to operational hazards, and
- Enable predictive maintenance and roller life cycle monitoring.
Conclusion
Results are very encouraging, showing that recent developments in IoT technology lend themselves to solving some of the most challenging mining industry problems.
LoRaWAN connectivity was a good option in this case, given that:
- It demands little energy for internal circuitry.
- It is inexpensive and has good penetration capabilities to work in harsh environments and even confined ones, like tunnels.
- LoRa is not IP-based, and only needs a concentration infrastructure (LoRa Gateways) at each 3 Km (average). Depending on the situation and roller quantities, the distances can be bigger. If necessary, on the other side, it’s not a problem to increase the spatial distribution by deploying more gateways in specific sites.
- LoRaWAN gateways can receive an average of 700,000 messages per day (with 8-channels), allowing for almost 5,000 rollers to send data every 10 minutes.
- LoRa operates on ISM frequencies and is possible to deploy private LoRaWAN networks, avoiding dependency from third party mobile or network operators in remote geography (most of the times).
- LoRaWAN is secure and allows for telemetry that is isolated from OT Network.
Resulting from this case study, other developments have been started to continue evolving the technology, namely:
- The development of an internal power generator to eliminate the need for batteries.
- The introduction of roller RPM and bearing vibration measurements, to detect roller shell wear and bearing predictive monitoring, respectively.
- Implementation of downlink messages that will allow to control some aspects of the roller, if necessary (like data sampling rate, firmware updates, test messages, and so on).
- Development of an improved heuristic to send telemetry data only when a given value threshold is reached, and not every 10 minutes whatsoever. This strategy brings more intelligence to the roller, saving unnecessary, repeated value messages, as well as energy, and bringing to the systems only readings that need more attention or action from the responsible team.
It is also clear from this case that, once widely adopted, the massive amounts of data generated by roller sensors will require large quantities of processing power to parse and analyze. Such processing power is currently only available at acceptable costs on large cloud farms. Development in that direction has also been started, including integration with Warehouse and Plant Maintenance systems to realize benefits since the purchase of the roller in a sustainable way.
This case study is by Ralf Luis de Moura, operational technology architecture, Vale; Alex Calegari Fracaroli, operational technology architecture, TCS; Danilo Bibancos, managing director, Superior Industries; Eduardo Martinelli, technology director, Superior Industries; Luiz Paulo Barreto, operational technology architecture, Vale
References
[1] G. Fedorko, et al. Failure analysis of belt conveyor damage caused by the falling material. Part I: Experimental measurements and regression models. Engineering failure analysis, 36, pp. 30-38, 2014.
[2] L. Zhao, Typical failure analysis and processing of belt conveyor. Procedia Engineering, 26, pp.942-946, 2011.
[3] M. Fiset; D. Dussalt. Laboratory simulation of the wear process of belt conveyor rollers. Wear, 162, pp. 1012-1015, 1993.
[4] Y. Guo, et al. Research on the idler spacing of belt conveyor. In: Applied Mechanics and Materials . Trans Tech Publications Ltd, pp. 295-299, 2012.
[5] A. Reicks, V. Allen. Belt conveyor idler roller behaviors. Bulk material handling by conveyor belt, 7,11, pP. 35-40, 2008.
[6] M. D. Jagtap; B. D. Gaikwad; P. M. Pawar. Study of roller conveyor chain strip under tensile loading. IJMER, 4, pp. 2249-6645, 2014.
[7] S. M. Shinde; R. B. Patil. Design and Analysis of a Roller Conveyor System for Weight Optimization and Material Saving. International Journal on Emerging Technologies, 3(25), 2012.
[8] M. L. A. Andrade and J. R. M. Vieira and L. M. S. Cunha and R. S. Fulda, “Minério de Ferro”, Mineraçao e Metalurgia, Out. 1997, pp. 1-8.
[9] M. Werner; et al. Desenvolvimento de uma bancada de teste de desgaste abrasivo para barras de trilha de colheitadeiras de grão: um comparativo de desempenho em acos SAE 1045 e SAE 1518. Centro de Pesquisas em Hidráulica e Recursos H´ıdricos, 2013.
[10] M. M. Loures. Sistema automático de deteccão de falhas em rolamentos de roletes de correias transportadoras,Universidade Federal de Minas Gerais, Escola de Engenharia, 2010.
[11] L. P. Ponci; P. M. R. Cunha. Previsão de falha em rolamentos por análise espectral e de envelope. Teknikao, pp. 1-11, 2005.
[12] Minipa Sense. Prevençao de incendios em correias de transporte. Jun. 2020. URL: http://www.minipasense.com.br/sensoriamento-termico-de-fibra-otica/prevencao-de-incendios-em-correias-de-transporte.
[13] Bosh. Solutions for the mining industry. Jun. 2020. Url: https://assets.bosch.com/media/global/products and solutions/market specific solutions/solutions for mining facilities/brochure.pdf.
[14] R. L. de Moura, L. F. Ceotto, and A. Gonzalez, “Industrial IoT and Advanced Analytics Framework: an approach for the Mining Industry. International Conference on Computational Science and Computational Intelligence, 2017.
[15] Dinamox. DinaPredict. Jun. 2020. URL: https://dynamox.net/.
[16] G. Garcia, et al. ROSI: A Novel Robotic Method for Belt Conveyor Structures Inspection. In: 2019 19th International Conference on Ad-vanced Robotics (ICAR)- IEEE, 2019. p. 326-331.
[17] SKF Group: Rolling Bearings Catalogue, 2018. p. 115 Table 2 URL: https://www.skf.com/binaries/pub12/Images/0901d196802809de-Rolling-bearings—17000 1-EN tcm 12-121486.pdf
[18] SKF Group: Bearing damage and failure analysis, p.36, 2017. URL: https://www.skf.com/binaries/pub12/Images/0901d1968064c148- Bearing-failures—14219 2-EN tcm 12-297619.pdf
[19] Energy Consumption Analysis of LPWAN Technologies and Lifetime Estimation for IoT Application, p.8, 2020. URL: https://www.mdpi.com/1424-8220/20/17/4794/pdf